[ad_1]
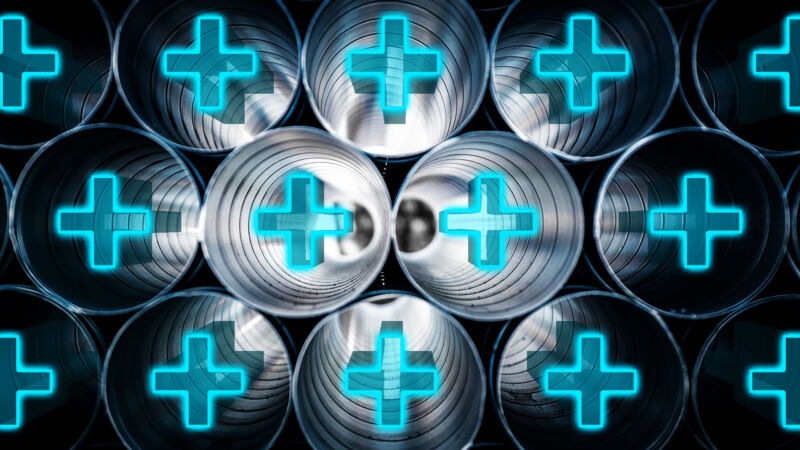
Orick Lawson | Getty Images
There’s a classic irony with new technology, that adopters are forced to limit themselves to two of the three things everyone wants: fast, cheap, and good. Adoption is more challenging when the technology is battery. Cheap and fast (charging) is still important, but “good” can mean different things, depending on your needs, such as light weight, low noise, or long lifespan. Still, the same trade-off is involved. If you need very fast charging, you may need to give up some capacity.
Despite lithium’s technological and manufacturing potential, research into alternative battery chemistries continues — there’s still hope that some other chemistries could offer a price cut or a big boost in some performance parameters.
Today, papers are being published that appear to offer low cost combined with large increases in many dimensions. The aluminum-sulfur batteries they describe offer lower-cost raw materials, competitive size and weight-to-weight capacity than lithium-ion — a big plus, fully charging cells in less than a minute. One obvious problem is that it must be at 90 degrees Celsius (boiling water) to work.
Is aluminum possible?
People are considering aluminum-based batteries, drawn by their high theoretical capacity. Although each aluminum atom is slightly heavier than lithium, aluminum atoms and ions are physically smaller because the nucleus’s higher positive charge pulls the electrons less. In addition, aluminum easily donates up to three electrons per atom, meaning you can exchange multiple charges for each ion.
The big problem is chemically, the type of aluminum is washed. Many aluminum compounds are very insoluble in water, their oxides are extremely stable, and so on – it’s easy for what could be a minor side reaction to cripple the battery after a few charge/discharge cycles. Therefore, although the work continues, high theoretical capabilities often seem to be practically unknown.
The key to the new work was the realization that we had solved one of the biggest problems in making aluminum metal electrodes – we did it in a completely different field. Pure metal electrodes offer a huge increase in lightness and volume, because there is no real chemistry involved and you don’t need additional materials to charge the metal ions. But metal tends to deposit unevenly on battery electrodes, eventually producing spines called dendrites that grow until they damage other parts of the battery or drain the cell entirely. So figuring out how to deposit iron evenly has become a major hurdle.
The key insight here is that we already know how to evenly deposit aluminum. We always do when we want to electroplate aluminum on another metal.
It is usually dissolved using aluminum chloride salt. In molten salt, alternating atoms of aluminum and chlorine ions form long chains. Aluminum tends to come out of the center of these chains when placed on the ground, and the remaining physical mass of the chain makes it easier to work on flat surfaces.
In the molten salt, aluminum ions can move rapidly from one electrode to the other. The biggest problem is that aluminum chloride only melts at 192°C. But mixing in a little sodium chloride and potassium chloride brought this down to 90°C – well below the boiling point of water and compatible with large amounts of additives.
Salt sandwich
With that, the researchers had two-thirds of the battery. One electrode was lithium metal, and the electrolyte was liquid aluminum chloride. This makes the second electrode known. Here, there were many examples of storing aluminum as a chemical compound. For imaging purposes, the team worked with selenium to create an experimental battery cell and found it performed as expected.
The aluminum image shows that after some charge and discharge cycles, the strip became somewhat blocked, but there were no large or pointed extensions from it that could damage the battery. It appeared that the reactions at the selenium electrode started in the molten salt before being completed at the electrode surface. Overall, the cell showed stable performance over dozens of cycles and the highest capacity per weight type that aluminum has to offer. So, the team proceeded to build and test the cells they were looking for: aluminum sulfur.
At slower speeds, aluminum sulfur cells had three times the charge capacity per mass of lithium-ion batteries. That figure decreased as the charge/flow rate increased, but performance was excellent. If the cell is discharged for more than two hours and recharged in just six minutes, it still has a charge-per-weight capacity 25 percent higher than lithium-ion batteries and retains 80 percent capacity after 500 cycles—more than you can afford. See in most lithium chemistry.
If you reduced the charging times to a little over a minute, the capacity per weight was equal to that of a lithium-ion battery, and more than 80 percent of the capacity was still available after 200 cycles. A battery cell can endure a full charge in less than 20 seconds, although the capacity per weight is more than half of what you get from lithium-ion.
[ad_2]
Source link